Handheld bioprinting is an innovation with huge potential, says Mohsen Akbari, a bioprinting engineer
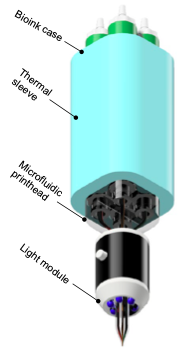

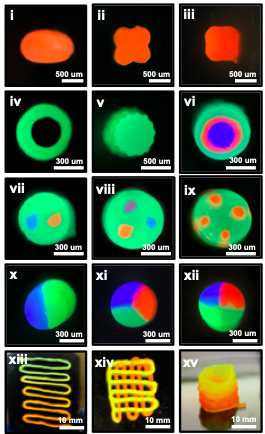
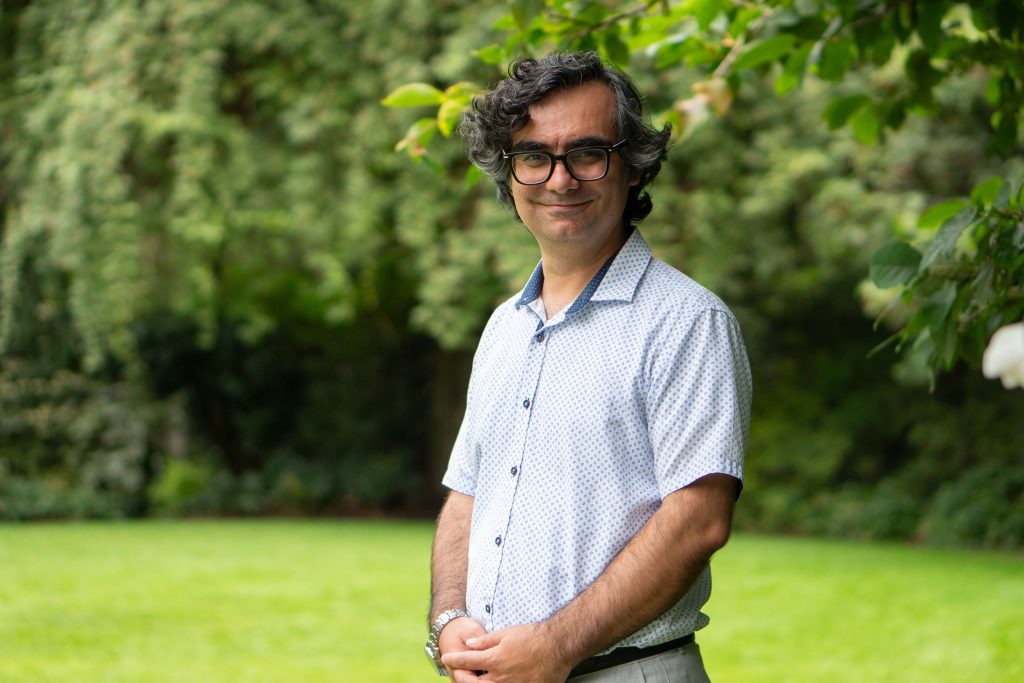
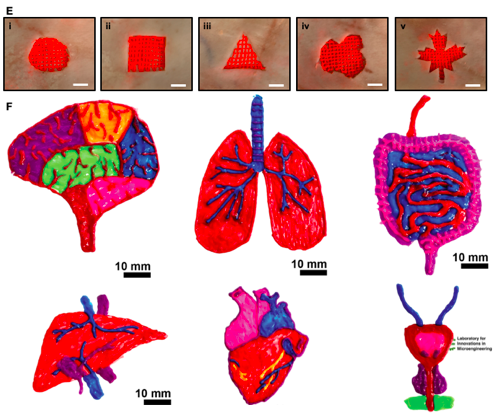
By Robert Price
The problems associated with using bioprinting to treat injuries are well documented. You can’t print larger-scale tissues because of the inherently small printing capability of bioprinters. You need to grow tissues in a bioreactor before they can be used to treat an injury. And once the tissues are grown, you need to transfer them to the site of the injury without contaminating the tissue and causing post-surgical infections. And another challenge looms large – clinicians will need to image an injury, wait a few weeks to prepare the tissue, and then print it.
“And during those first few weeks of the injury, the size and shape will most probably change, right? Because the body is a dynamic environment, and then you have to redo everything again or find a way to fit this tissue into the body.”
So explains Mohsen Akbari, a veteran of the field of bioprinting for the past ten years. Currently an associate professor at the University of Victoria with affiliations with the University of British Columbia and the University of California, Irvine, Akbari plays a major role in two spin-off companies from his lab: 4M Biotech, a business developing smart wound dressing, and Apricell Biotechnology, a startup developing next generation tumour-on-a-chip technologies.
Faced with all the challenges related to treating injuries with bioprinted materials, a few groups started thinking about directly printing cells and materials at the site of injury. One group in Australia came up with the idea of moving the printheads of the bioprinter to the injury site and asking the surgeon to print directly onto the wound—an idea that eliminated the need to transport the tissues to the body after growing them.
“The thing is that we don’t need to have those bioreactors to grow the cells,” explains Akbari. “The body will act as a bioreactor itself and provide the suitable environment for these cells to grow and remodel the thing that forms.”
Many groups started developing this idea, but they faced a new challenge: the devices that they made were only compatible with one type of photocurable material. When photocurable materials are exposed to ultraviolet light, the material cures directly at the deposition site. The problem is that not all cells are compatible with photocurable materials, and because these materials need light and photo initiators that can become toxic at high concentrations, they can lead to carcinogenic issues.
Along with these significant challenges, researchers in this field have to deal with the fact that the body is made out of many types of cells, and injuries may require several cell types.
“You want to be able to deliver multiple cells and multiple materials with different mechanical properties, chemical properties, to the site of the injury to be able to mimic the complexities of the tissues in the body,” says Akbari.
Unfortunately, older bioprinters did not have such capabilities. That’s where Akbari saw an opportunity—the creation of a handheld bioprinter.
Handheld device
Bioprinters print in a three-dimensional space, primarily using biological materials like cells. These cells are encapsulated in a medium made mostly out of water and proteins like collagen, gelatine, and hyaluronic acid typically found in the body that mimics the conditions that the cells experience.
Handheld bioprinters, like the one Akbari is developing, enables researchers and clinicians to create three-dimensional structures in situ, directly at the point of care, directly at the site of injury and disease.
Handhelds bioprinter looks like a stylus, or a pen. Akbari’s stylus is a microfluidic device with a computer-controlled system that allows the user to deliver multiple materials sequentially or simultaneously to the site of the injury and change the ink that’s being printed.
“This means that we would like to be able to, while you’re using this printer, change materials on the fly without any need to change the pen itself,” says Akbari.
This is a useful development. Right now, with current printers, if users want to change the material, they have to change the pen to print or to write different inks.
The way Akbari and his colleagues accomplished this innovation was by using 3D resin printing to create microfluidic devices that look like a pen connected to different cartridges. By applying hydraulic pressure to each of these cartridges, a user can deliver controlled amounts of different materials to the site of the injury.
The technology can print, for example, fat cells at the bottom of the injury, fibroblast cells on top of the fat cells, and keratinocytes on top of that until the entire cavity of the injury is filled.
“And then, of course, the injury will be covered and then the cells start interacting with each other until the injury is healed,” says Akbari.
One of the unique features of Akbari’s technology is the fact that it uses microfluidic printers. These allow the user to manipulate different types of flows and generate different structures and a variety of geometries, including hollow and luminal shapes, when the printer deposits the materials in strands of fibres.
In a recent paper, Akbari and his colleagues demonstrated that they can print hollow tubes. These hollow tubes can be perfusable, meaning they can connect to one another. The researchers haven’t done that yet but hope to connect them to the vasculature.
The tubes are made out of gels that the researchers can seed with cells and use to mimic these endothelial layers and blood vessels typically seen in the body.
“And with that, we can bypass the issue of vascularisation, which is a major issue in the field of tissue engineering,” says Akbari.
A vision
One of the visions driving Akbari is to create cells using. The process will involve collecting skin cells or blood cells and using now well-established protocols to reprogram those cells into induced pluripotent stem cells. Under the right conditions, induced pluripotent stem cells can be differentiated into any type of cells in the body. Akbari plans to mix keratinocytes, fibroblasts, and fat cells from induced pluripotent stem cells with ingeniac biomaterials (bioinks) to deliver the cells directly to the site of injury.
But there are a number of barriers standing between Akbari and his vision. First, the device itself has to be validated by surgeons. “They are going to be the end users of this technology,” says Akbari.
The major barrier to regulatory approval is safety. The device itself is made from materials that are compatible with the human body, but the materials the pen prints need to be safe to humans.
“Stem cells, they can differentiate into any cells, and one of the cells that they can differentiate into is cancer cells, right? They just want to make sure that you don’t want to form tumours,” says Akbari.
Challenges like this one posed by stem cells are currently being addressed by other research groups. For now, Akbari and his colleagues will have to wait until issues related to materials are solved before they will see their technology used in clinics.
In the meantime, Akbari and his colleagues are thinking about other applications for this innovation. One is to use the handheld bioprinter in the visual arts to mix different materials and different inks without pausing to change cartridges and colours.